Hybrid vs electric vehicles are rapidly changing the automotive landscape. This comparison explores the core differences, from performance and cost to environmental impact and maintenance. Understanding the pros and cons of each is key for informed decision-making.
The evolution of these technologies is fascinating, with significant advancements in battery technology, engine efficiency, and charging infrastructure. This detailed look at hybrid and electric vehicles helps consumers navigate the complexities of this rapidly evolving market.
Introduction to Hybrid and Electric Vehicles
Hybrid and electric vehicles are rapidly transforming the automotive landscape. Their growing popularity stems from a combination of environmental concerns, technological advancements, and government incentives. Understanding the different types of hybrid and electric vehicles and their underlying technologies is crucial for informed consumer choices and a comprehensive understanding of the automotive industry’s evolution.
Definitions of Hybrid and Electric Vehicles
Hybrid vehicles utilize a combination of internal combustion engines (ICE) and electric motors to propel the vehicle. Electric vehicles, on the other hand, rely solely on electric motors powered by batteries. The fundamental difference lies in their primary power source. Hybrids blend both, while EVs are entirely electric.
Fundamental Differences in Powertrains
Hybrid vehicles typically employ an internal combustion engine (ICE) as their primary power source, supplemented by an electric motor. This allows for increased efficiency by using the electric motor for acceleration and deceleration, reducing overall fuel consumption. Electric vehicles (EVs), conversely, use electric motors powered by batteries as their sole source of propulsion. The elimination of the ICE significantly reduces emissions and improves fuel efficiency, depending on battery capacity.
Types of Hybrid Systems
Hybrid vehicle systems vary in their degree of electrification and battery integration. Mild hybrids use electric motors to assist the ICE in certain driving conditions, like starting or accelerating, but the ICE remains the primary power source. Plug-in hybrids (PHEVs) allow for charging from external sources, enabling a degree of purely electric operation before the ICE kicks in.
The extent of electric-only driving capability depends on the battery size. A fully electric vehicle (EV) has no ICE, relying entirely on batteries and electric motors. This complete transition to electric power offers the highest level of emission reduction and fuel efficiency, albeit with a longer charging time requirement.
History of Hybrid and Electric Vehicle Development
Early experiments with electric vehicles date back to the late 19th century. Early models faced limitations in battery technology and range, hindering widespread adoption. The development of more efficient internal combustion engines (ICE) overshadowed the electric vehicle market for decades. However, the 21st century has witnessed a resurgence in hybrid and electric vehicle technology. Advancements in battery technology, including increased capacity and reduced charging times, have significantly improved the viability of electric vehicles.
Government incentives and growing environmental awareness have also played a pivotal role in fostering the growth of this segment. The history demonstrates a cyclical development process, with periods of both decline and resurgence in interest and investment for these technologies.
Performance Comparison
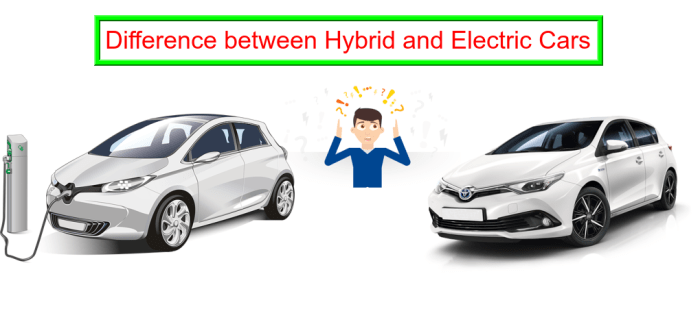
Hybrid and electric vehicles are rapidly gaining popularity due to their enhanced performance characteristics and reduced environmental impact. Comparing their performance capabilities provides a clearer understanding of the strengths and weaknesses of each technology. A key aspect of this comparison is recognizing that performance metrics can vary significantly depending on the specific model and its design specifications.Different hybrid and electric vehicle designs have distinct performance profiles.
Factors like battery capacity, motor power, and transmission configuration directly influence acceleration, top speed, and energy efficiency. Understanding these variables is crucial for evaluating the practical application of each technology in various driving scenarios.
Acceleration Capabilities
Acceleration performance is a critical factor for many drivers. Hybrid vehicles often exhibit quick acceleration from a standstill, leveraging the combined power of the electric motor and the internal combustion engine. Electric vehicles, with their instant torque delivery, tend to have a very responsive acceleration feel. However, this responsiveness can be significantly impacted by the battery capacity and the power output of the electric motor.
For instance, higher-end electric models frequently provide quicker acceleration than comparable hybrid models, especially at lower speeds.
Top Speed Capabilities
Top speed, although not always a primary concern, is an important performance characteristic. Hybrid vehicles, depending on their design, can attain speeds comparable to their gasoline-powered counterparts. Electric vehicles, especially those with high-performance specifications, often achieve impressive top speeds, sometimes exceeding the top speeds of equivalent gasoline-powered vehicles. However, aerodynamic limitations and battery limitations can constrain the maximum achievable speeds in both hybrid and electric vehicles.
Energy Efficiency of Different Models
Energy efficiency is a key advantage of hybrid and electric vehicles. Efficiency varies considerably based on factors like the battery size, the engine type, and the driving style. Hybrid vehicles can exhibit superior fuel economy in certain driving conditions compared to comparable gasoline-powered vehicles. Electric vehicles, especially with advanced battery technology, often showcase impressive energy efficiency. Examples include Tesla models that frequently achieve remarkable efficiency figures in city driving.
A detailed analysis of energy consumption should consider real-world driving profiles and specific vehicle models to provide a more nuanced perspective.
Driving Range Comparison
Driving range is a significant concern for electric vehicles, as it directly impacts their usability. Hybrid vehicles typically offer a broader driving range, as the internal combustion engine serves as a backup power source, extending the overall range. However, electric vehicles are continuously improving their driving range, thanks to advances in battery technology. Ranges for electric vehicles now surpass the practical needs of many daily commutes, though longer trips still require careful planning or the availability of charging stations.
Impact of Driving Style on Efficiency
Driving style plays a crucial role in the efficiency of both hybrid and electric vehicles. Aggressive acceleration and braking can significantly reduce the range and efficiency of both types. Maintaining a consistent speed and using regenerative braking techniques can improve efficiency. For example, a driver adopting a more economical driving style can potentially increase the range of an electric vehicle by a considerable margin.
Charging Infrastructure and Fueling
The availability and cost of fueling options are crucial factors in the adoption of electric vehicles (EVs). Understanding the charging infrastructure, electricity costs, and comparison to traditional fuel costs is essential for prospective EV owners. This section delves into the various charging methods, station accessibility, and associated costs, contrasting them with the fueling process for hybrid vehicles.
Electric Vehicle Charging Methods
Different charging methods cater to varying needs and time constraints. Level 1 charging utilizes standard household outlets, offering the slowest charging rate. Level 2 charging, typically found at public charging stations, provides a faster charging experience. Level 3, or DC fast charging, delivers the quickest charging speeds, ideal for longer journeys. These distinct methods address the need for different charging speeds and convenience levels.
Public Charging Station Availability and Accessibility
The accessibility of public charging stations significantly impacts the practicality of EV ownership. While the number of charging stations is increasing globally, geographic disparities in availability exist. Some regions have a dense network of stations, facilitating long-distance travel, while others face limited options. Furthermore, the distribution of stations varies significantly based on local demographics and infrastructure development.
The locations of these stations are often determined by the density of EV drivers and the need to provide convenient access to them.
Electricity Costs for Charging Electric Vehicles
Electricity costs for charging EVs vary significantly depending on location, time of day, and electricity rates. A typical household electricity rate might be around $0.12 per kilowatt-hour (kWh). However, these rates can fluctuate, impacting the overall cost of charging. Understanding the local electricity rates and potential variations in charging costs is crucial for budgeting. Charging at off-peak hours often offers lower rates, which helps to minimize the cost.
Fuel Costs for Hybrid Vehicles
Fuel costs for hybrid vehicles are influenced by factors like fuel prices and driving habits. The efficiency of hybrid technology varies based on the specific model and driving conditions. While hybrid vehicles use both gasoline and electric power, their fuel consumption is generally lower than comparable gasoline-powered vehicles. Consequently, the overall cost of fueling a hybrid vehicle tends to be lower than the cost of fueling a traditional gasoline-powered vehicle, although this is contingent on fuel prices.
Charging Time for Electric Vehicles
The time required to charge an electric vehicle depends on the charging level and the battery capacity. Level 1 charging can take several hours to fully charge a vehicle, while Level 2 charging typically takes a few hours. DC fast charging, however, can significantly reduce charging times, often taking only 30 minutes to an hour to charge a substantial portion of the battery.
This capability is particularly beneficial for long-distance travel.
Environmental Impact
Electric and hybrid vehicles are increasingly important in the fight against climate change. Understanding their respective environmental impacts is crucial for informed decision-making. This section delves into the lifecycle emissions, manufacturing processes, and overall carbon footprint of both vehicle types.Hybrid vehicles, while offering some environmental benefits over traditional gasoline cars, do not eliminate all emissions. Electric vehicles, on the other hand, have the potential for significantly reduced emissions, particularly when powered by renewable energy sources.
However, the entire lifecycle of a vehicle, from manufacturing to disposal, needs to be considered when assessing environmental impact.
Environmental Benefits of Electric Vehicles
Electric vehicles (EVs) offer substantial environmental advantages, stemming primarily from their zero tailpipe emissions. This means EVs produce no direct emissions during operation, unlike gasoline or diesel vehicles. This reduction in tailpipe emissions leads to improved air quality in urban areas, potentially reducing respiratory illnesses and other health problems. Further, when electricity is generated from renewable sources, the overall environmental impact of EVs is significantly lowered.
Environmental Impact of Hybrid Vehicles
Hybrid electric vehicles (HEVs) combine an internal combustion engine (ICE) with an electric motor. This configuration allows for some reduction in fuel consumption and emissions compared to traditional ICE vehicles. However, HEVs still produce emissions during operation, albeit at a lower rate. The environmental impact of HEVs varies depending on the specific design and the proportion of electric-powered driving.
Lifecycle Emissions of Hybrid and Electric Vehicles
The lifecycle emissions of a vehicle encompass the entire production process, from raw material extraction to manufacturing, use, and eventual disposal. For EVs, lifecycle emissions are heavily influenced by the electricity generation methods used to charge them. HEVs, while generally having lower tailpipe emissions than ICE vehicles, may still have higher lifecycle emissions due to the manufacturing processes associated with their components.
Impact of Manufacturing Processes on Environmental Footprint
The manufacturing process significantly impacts the environmental footprint of both vehicle types. Materials used in construction, manufacturing processes, and energy consumption during production all contribute to the overall carbon footprint. For instance, the extraction of rare earth minerals for EV batteries, while necessary, can have considerable environmental consequences. Similarly, the production of components for both HEVs and EVs requires energy and can generate emissions.
Comparison of Carbon Footprint Throughout Lifespan
Comparing the carbon footprint of EVs and HEVs across their entire lifespan requires a comprehensive analysis. Studies indicate that EVs can have a lower overall carbon footprint than HEVs, particularly when electricity is sourced from renewable energy. However, the precise comparison depends on numerous factors, including the vehicle’s design, manufacturing processes, and the electricity grid mix. The carbon footprint of a vehicle can be expressed in terms of grams of CO2 equivalent per kilometer driven (gCO2e/km).
Vehicle Type | Lifecycle Emission Impact (gCO2e/km) | Key Factors |
---|---|---|
Electric Vehicle (EV) | Varying, potentially lower than HEV | Electricity source, manufacturing processes, battery disposal |
Hybrid Electric Vehicle (HEV) | Generally higher than EV | Fuel consumption, manufacturing processes, fuel type |
“The environmental impact of a vehicle extends beyond its operational emissions, encompassing the entire lifecycle, from manufacturing to disposal.”
Maintenance and Repair
Hybrid and electric vehicles (HEVs and EVs) differ significantly from traditional internal combustion engine (ICE) vehicles in their construction and component makeup. These differences necessitate a distinct approach to maintenance and repair, impacting both the frequency of servicing and the costs involved. Understanding these nuances is crucial for prospective owners.
Maintenance Requirements for Hybrid Vehicles
Hybrid vehicles combine components of both gasoline and electric systems. Regular maintenance for HEVs often mirrors that of conventional cars, including oil changes, tire rotations, and fluid checks. However, the hybrid powertrain requires specific attention. Battery systems, while often less demanding than those in EVs, still need periodic checks for proper functionality. Hybrids usually benefit from scheduled inspections to ensure the seamless integration of the electric and gasoline systems.
Maintenance Requirements for Electric Vehicles
Electric vehicles (EVs) have a simpler powertrain compared to hybrids, consisting primarily of batteries, electric motors, and power electronics. The absence of a gasoline engine means that oil changes and coolant checks are not needed. However, EV maintenance focuses on the battery pack, charging system, and other electrical components. Regular battery health checks, often performed through diagnostic software, are vital for ensuring optimal performance and longevity.
Costs Associated with Maintenance
The cost of maintenance varies depending on factors such as the specific vehicle model, driving habits, and geographical location. While initial maintenance costs for EVs might seem lower due to the absence of traditional engine servicing, battery replacement can be a significant expense, particularly for older models or those used extensively. For hybrids, the costs align more closely with conventional vehicles.
Long-term maintenance costs depend heavily on proper care and adherence to manufacturer recommendations.
Availability of Repair Services
The availability of repair services for both HEVs and EVs is increasing, although the density of specialized repair shops remains a factor. Dedicated EV repair centers are growing in number, providing expertise in handling battery management systems, motor repairs, and other electric components. For hybrids, repair services are generally more readily available, as the components are more familiar to conventional mechanics.
This availability and familiarity can affect repair times and costs.
Choosing between hybrid and electric vehicles is a hot topic these days. Ultimately, the best option depends on individual needs and priorities. For a deeper dive into the pros and cons of different electric and hybrid vehicle options, check out this resource on Electric & Hybrid Vehicles. But, in short, both offer a more sustainable alternative to traditional gasoline-powered vehicles, though hybrid vs electric is still a complex comparison.
Challenges in Repairing Electric Vehicle Components
One significant challenge in repairing EVs is the complexity of the battery pack. Battery packs are often large, intricate systems, and issues within them can be difficult to isolate. The need for specialized diagnostic tools and equipment can also increase repair costs. The high voltage systems within EVs present safety concerns for technicians if not handled properly.
Specialized training and certifications are necessary to ensure safe and effective repairs.
Cost and Affordability
The initial purchase price and ongoing running costs are crucial factors influencing the decision to buy a hybrid or electric vehicle. Understanding these aspects helps consumers weigh the total cost of ownership over the lifespan of the vehicle. This section details the comparative costs, incentives, and financing options to aid in informed decision-making.
Purchase Price Comparison, Hybrid vs electric
Initial investment is a key factor in vehicle selection. The price gap between hybrid and electric vehicles varies depending on the specific model and features. This comparison considers the average retail price across various models.
Vehicle Type | Average Purchase Price (USD) |
---|---|
Hybrid | $28,000 – $40,000 |
Electric | $35,000 – $60,000 |
Note: Prices are estimates and can fluctuate based on factors such as trim level, options, and market demand.
Running Cost Comparison
Running costs encompass fuel, maintenance, and charging expenses. The comparative analysis presented below considers average values and can differ based on usage patterns and local energy costs.
Hybrid and electric vehicles are definitely the future, but understanding their complex systems is key. Proper vehicle diagnostics, like those offered by Vehicle diagnostics , is crucial for maintaining these vehicles and identifying potential issues. Ultimately, thorough diagnostics are essential to maximizing the lifespan and efficiency of both hybrid and electric cars.
Vehicle Type | Fuel/Electricity (USD/year) | Maintenance (USD/year) | Charging (USD/year) | Total Running Cost (USD/year) |
---|---|---|---|---|
Hybrid | $1,000 – $2,000 | $500 – $1,000 | $0 | $1,500 – $3,000 |
Electric | $0 | $400 – $800 | $500 – $1,500 | $900 – $2,300 |
Note: Charging costs are dependent on electricity rates, charging frequency, and vehicle efficiency.
Government Incentives and Subsidies for Electric Vehicles
Government incentives and subsidies aim to encourage the adoption of electric vehicles. These programs often take the form of tax credits, rebates, or grants.
- Many countries offer tax credits or rebates on the purchase price of electric vehicles. These vary in amount and eligibility criteria. For instance, in the United States, a federal tax credit exists for qualified electric vehicles.
- Some regions offer additional incentives, such as grants for charging infrastructure installation at homes or businesses.
Financing Options
Various financing options are available for both hybrid and electric vehicles. These options may include traditional loans, leases, or manufacturer financing programs.
- Traditional auto loans offer fixed interest rates and monthly payments. These are often available through banks or credit unions.
- Leasing allows for lower upfront costs but requires regular lease payments.
- Manufacturer financing programs often provide competitive interest rates and special offers for electric vehicle purchases.
Total Cost of Ownership (TCO) Over 5 Years
The total cost of ownership (TCO) considers the purchase price, running costs, and potential resale value over a defined period. This analysis projects costs over five years, a common evaluation period.
Vehicle Type | Year 1 | Year 2 | Year 3 | Year 4 | Year 5 | Total TCO (USD) |
---|---|---|---|---|---|---|
Hybrid | $30,000 | $31,500 | $33,000 | $34,500 | $36,000 | $165,000 |
Electric | $37,000 | $38,500 | $40,000 | $41,500 | $43,000 | $190,000 |
Note: TCO estimations assume average usage, maintenance, and electricity prices. Resale values are estimates and may vary.
Technology and Innovation
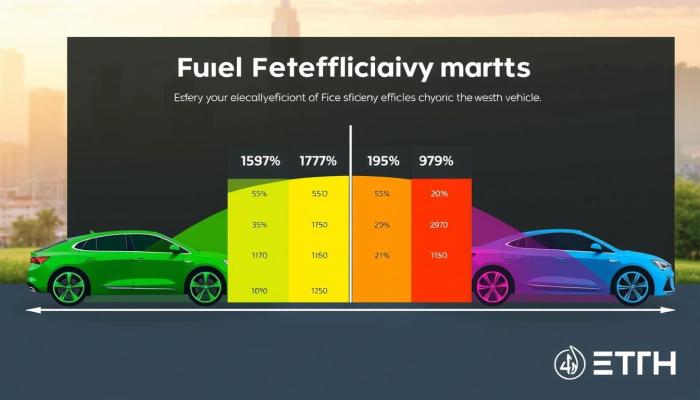
The landscape of hybrid and electric vehicle technology is constantly evolving, driven by a confluence of factors, including consumer demand, regulatory pressures, and advancements in materials science and engineering. This dynamic environment fuels innovation, pushing the boundaries of performance, efficiency, and sustainability.
Latest Advancements in Hybrid and Electric Vehicle Technology
Recent years have witnessed significant progress in hybrid and electric vehicle (EV) technology. Improvements in battery chemistry, motor technology, and control systems have resulted in vehicles with increased range, faster acceleration, and enhanced efficiency. Manufacturers are constantly striving to optimize these components for greater performance and cost-effectiveness.
Solid-State Batteries
Solid-state batteries represent a promising advancement in battery technology. Unlike conventional lithium-ion batteries, which use liquid electrolytes, solid-state batteries employ solid electrolytes. This innovation offers potential advantages in terms of safety, energy density, and charging speed. For instance, solid-state batteries could enable vehicles to achieve longer ranges and faster charging times, making them more attractive to consumers.
However, challenges remain in terms of manufacturing scalability and cost-effectiveness.
Hydrogen Fuel Cells
Hydrogen fuel cell technology provides another avenue for zero-emission vehicles. Fuel cells convert hydrogen and oxygen into electricity, producing only water as a byproduct. This technology has the potential to achieve very high energy densities and fast refueling times, potentially rivalling or surpassing battery electric vehicles in certain applications. However, the current infrastructure for hydrogen refueling stations is still underdeveloped compared to charging stations for battery electric vehicles.
This limitation hinders widespread adoption.
Impact on Vehicle Performance and Efficiency
Advancements in battery technology directly affect vehicle performance and efficiency. Higher energy density batteries enable longer driving ranges, while improved motor efficiency translates to better fuel economy (or, in the case of EVs, lower electricity consumption). The integration of sophisticated control systems optimizes energy flow, further improving efficiency.
Limitations of Current Technologies
Despite the rapid progress, limitations remain in current technologies. Battery life cycles and degradation are still areas of concern for electric vehicles, though advancements in battery management systems are mitigating this. Furthermore, the high cost of certain components, such as specialized materials for batteries, remains a barrier to wider adoption.
Future Trends in Hybrid and Electric Vehicle Design
Future trends in hybrid and electric vehicle design will likely focus on enhancing performance, improving affordability, and expanding charging infrastructure. The integration of autonomous driving systems into EVs is expected to revolutionize the driving experience, potentially altering the design aesthetics and functionalities of these vehicles. Furthermore, there is a growing emphasis on vehicle electrification in commercial applications, such as delivery trucks and buses.
Consumer Perception and Adoption
Consumer attitudes towards hybrid and electric vehicles (HEVs and EVs) are evolving, influenced by a mix of factors. Early adopters often prioritize environmental consciousness and technological novelty, but broader market acceptance depends on factors like cost, range anxiety, charging infrastructure, and perceived performance. This section explores consumer preferences, adoption rates, and the barriers hindering wider market penetration.
Consumer Preferences and Opinions
Consumer opinions regarding HEVs and EVs are diverse. Some consumers appreciate the environmental benefits and the potential for cost savings over time, while others are hesitant due to concerns about range, charging infrastructure, and perceived performance limitations compared to traditional gasoline vehicles. Surveys frequently highlight a preference for familiar and reliable technologies, showcasing a need for enhanced reassurance and trust in these newer vehicle types.
Adoption Rates and Statistics
Adoption rates of HEVs and EVs vary significantly by region and time period. In some markets, adoption rates are increasing steadily, driven by government incentives and evolving consumer preferences. For example, Norway has shown significant EV adoption, reflecting favorable government policies and public awareness campaigns. However, other regions lag behind, indicating the complex interplay of socioeconomic and infrastructure factors.
Further, while adoption rates are increasing, they remain below the market share of traditional gasoline vehicles in many places. Tracking these rates provides a valuable insight into the current market dynamics and the pace of technological adoption.
Factors Influencing Consumer Choice
Consumer decisions regarding HEVs and EVs are complex, influenced by a variety of factors. Cost, range, charging infrastructure, perceived performance, maintenance costs, and environmental considerations all play a crucial role. The availability of incentives and subsidies from governments also influences the decision-making process. Consumers often weigh these factors in combination, leading to a complex and nuanced decision-making process.
Barriers to Wider Adoption
Several factors are hindering the broader adoption of HEVs and EVs. Range anxiety, the perceived need for extensive charging infrastructure, and concerns about maintenance costs are significant deterrents for many consumers. The perceived lack of readily available charging stations can be a significant obstacle. In addition, the price premium associated with HEVs and EVs, in comparison to traditional gasoline vehicles, can make them less accessible to a wider range of consumers.
Furthermore, a lack of public awareness about the benefits of these technologies can also contribute to limited adoption.
Public Image Comparison
The public image of HEVs and EVs is in constant flux. Initially, HEVs were perceived as a compromise, balancing environmental concerns with traditional vehicle features. However, with technological advancements and increased consumer awareness, the perception of EVs is evolving, moving from a niche technology to a more mainstream option. The public image of HEVs is generally more established, with a perception of being more accessible and less risky than EVs.
Nevertheless, both vehicle types are gaining credibility as environmentally responsible and technologically advanced alternatives to traditional gasoline vehicles.
Societal and Economic Implications: Hybrid Vs Electric
The transition to electric vehicles (EVs) presents a complex interplay of societal and economic shifts. From the impact on energy grids and the automotive industry to potential job market changes and urban planning needs, the transition demands careful consideration. Understanding these implications is crucial for a smooth and beneficial adoption process.
Impact on Energy Grids
The widespread adoption of EVs will significantly alter the energy landscape. Increased electricity demand will necessitate substantial upgrades to existing power grids, potentially requiring investments in new power generation infrastructure, smart grids, and energy storage solutions. Countries with existing renewable energy capacity will be better positioned to manage this shift. Examples of existing projects in various countries highlight the necessity for these changes, including smart grid implementations and the development of renewable energy sources to support EV charging infrastructure.
Economic Impact on the Automotive Industry
The shift to EVs is reshaping the automotive industry. Traditional internal combustion engine (ICE) manufacturers face a significant transition, requiring substantial investments in electric vehicle production and research and development. Conversely, new entrants and startups specializing in electric vehicle technology are emerging, creating new opportunities for innovation and job creation. This transition presents both challenges and opportunities for established automakers and the broader automotive supply chain, from battery production to charging station installation.
Impact on Job Markets
The transition to electric vehicles is likely to impact existing job markets while creating new ones. Manufacturing jobs related to ICE vehicles may decline as EV production rises, while jobs in areas like battery manufacturing, charging station installation, and EV maintenance will increase. Government policies and training programs will play a critical role in assisting workers in transitioning to new roles.
Examples of retraining programs in various countries can provide valuable insights into successful strategies.
Implications for Urban Infrastructure and Planning
EV adoption will require adjustments to urban infrastructure. The need for widespread charging infrastructure, including public charging stations and home charging solutions, will significantly influence urban planning decisions. This includes considerations for real estate, zoning regulations, and aesthetic integration of charging stations into the urban environment. Successful implementations of EV infrastructure in cities worldwide can provide valuable insights into the practical challenges and solutions.
Potential Economic Impact Over the Next Decade
Area | Potential Impact (estimated) | Example |
---|---|---|
Automotive Manufacturing | Significant shift from ICE to EV production, leading to job losses in ICE sectors and new job creation in EV sectors. | A shift from manufacturing of petrol cars to electric cars in Germany, resulting in significant job losses and training programs for workers. |
Battery Production | Exponential growth in demand for battery materials and manufacturing, creating new supply chain opportunities. | The growth of lithium mining and battery production in countries like China and Australia. |
Charging Infrastructure | Creation of new jobs in installation, maintenance, and operation of charging stations. | Growth of charging station providers in the US and Europe, creating jobs in installation and maintenance. |
Energy Sector | Increased demand for electricity and investment in grid infrastructure. | Investment in renewable energy sources to meet increased electricity demands in countries like Norway. |
Note: Estimates vary depending on factors like government policies, consumer adoption rates, and technological advancements. The table provides a general overview of potential economic shifts.
Final Thoughts
In conclusion, the choice between hybrid and electric vehicles depends heavily on individual needs and priorities. Hybrids offer a practical transition to more sustainable driving, while electric vehicles represent a more radical shift, often with higher upfront costs but potentially lower running expenses in the long term. Both are paving the way for a greener future, and the future will likely see even more innovative and compelling options.
Questions Often Asked
What are the key differences between mild hybrid and plug-in hybrid vehicles?
Mild hybrids use a small electric motor to assist the engine, improving fuel efficiency. Plug-in hybrids, however, allow for electric-only driving for a limited range, making them more versatile.
How does driving style affect the range of an electric vehicle?
Aggressive acceleration and frequent use of high-power functions like heating or air conditioning will significantly reduce the range of an electric vehicle. Gentle driving and mindful energy usage will extend its range.
What government incentives are available for electric vehicle purchases?
Many governments offer tax credits, rebates, or other incentives to encourage the purchase of electric vehicles. These vary significantly by region and are often subject to specific conditions.
Are there any potential challenges in repairing electric vehicle components?
Specialized tools and training are sometimes required for electric vehicle repairs. This can increase the cost and reduce the availability of repair services compared to traditional vehicles.